or start from open source methods. Learn more about OneLab softwareUse OneLab
Simple Plate Reformatting
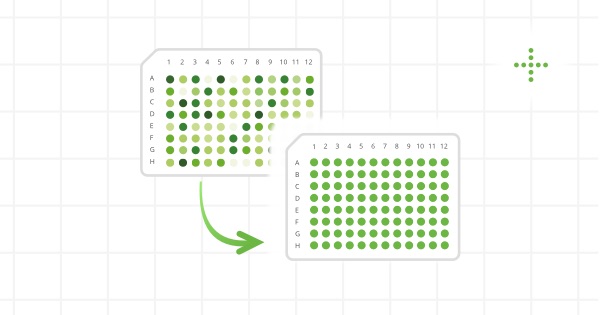
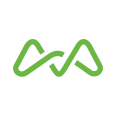
This example method provides a freely adjustable framework for measuring the adaptability of the OneLab environment to workflows from different application fields. It helps to understand various nuances of the code-free, universal protocol designer and provides general indications as to the feasibility of a project. Scripts generally require fine adjustment to correct for variables and support specific labware implementation.
Overview
Simple Automated Plate Reformatting using OneLab
Microplates are the consumable of choice for sample processing, high throughput screening, and storage in many fields of application including cell biology, drug discovery, genetics, immunology, in vitro diagnostics, etc... They played a pivotal role in the miniaturization of assay formats, minimizing the amount of precious reagents and compounds required, thereby enabling their use in a more cost-effective way. However, miniaturized assays are not always compatible with all microplates formats. Some assays are specifically designed for use with 96-well microplates while other applications require microplates with higher density. Therefore, a plate reformatting step is required, ensuring optimal performance of the assay.
Plate reformatting consists of transferring samples or compounds from one source microplate format to a destination plate with a different format. Plate reformatting usually involves a simple transfer of the same volume from one or multiple sources (partial or full) into one destination multi-well plate with higher or lower density, but can also involve more complex transfer from multiple sources to multiple destinations at different volumes. Further complexity can occur when replicates of each sample are required. As the task's complexity increases, so do the amount of hands-on time required and the risks to introduce errors and variability through inconsistent pipetting. Therefore, it becomes crucial to ensure rapid, yet efficient sample or compound transfer from one format to another, thereby minimizing pipetting errors and increasing productivity.
The Andrew+ Pipetting Robot is a versatile platform to easily automate routine pipetting activities, in particular plate reformatting. Andrew+ and the intuitive, Cloud-based OneLab software can efficiently accommodate any reformatting strategy using your lab consumables, ensuring precise pipetting and full traceability.
Plate Reformatting Modes
There are two plate reformatting modes, each including different strategies: Scaling or Custom Pattern.
Scaling Mode
The scaling mode comprises three reformatting strategies as described below:
- Compression: to compress partial or full 96-well plates into 384-well destination plate(s).
- Decompression: to decompress partial or full 384-well plates into 96-well destination plate(s).
- Replication: to replicate a partial or full 96-well or 384-well plate.
Custom Pattern Mode
Reformatting methods within the custom pattern mode include:
- Region transfer: Transfer any region in the source plate to any wells in the destination plate at any volume. The number of wells to transfer from the source plate must match the number of destination wells selected.
- Region replication: Replicate a region of the source plate into multiple regions in the destination plate.
- Interleaved transfer: Transfer samples from a single region in the source plate to interleaved regions in the destination plate.
- Pooling: Transfer samples from different source plates or different regions of the same source plate to the same region in the destination plate.
- Transposing: Change the orientation of the transferred region, for instance, from a horizontal to a vertical arrangement. The number of wells to transfer from the source plate must match the number of destination wells selected.
◼️ PROTOCOL 1 | Plate Reformatting - Compound Library
The example reformatting protocol #1 demonstrates the transfer of bioactive compounds from four different 96-well source plates (full) into interleaved regions in a 384-well destination plate as shown in Figure 2. Each source (compound) plate is transferred to 96 destination wells interleaved. The interleaved pattern is obtained by column-wise spacing using an 8-channel pipette.
Figure 1: Andrew+ Domino configuration for the protocol "Plate Reformatting - Compound Library" (left) and experiment setup comparison between Andrew+, Pipette+, and manual pipetting (right). Domino positions on the Andrew+ working deck: [1-5] Tip Insertion System Domino (10-300 µL tips), [6] Microplate Domino, [7-11] Deepwell Microplate Domino. The time required for Andrew+ to complete the protocol is ~30 min using an 8-channel 300 µL electronic pipette.
Figure 2: Schematic of the interleaved transfer of compounds from four 96-well source plates to a 384-well destination plate for compound management applications. The zoomed image of "A1 wells" in the 384-well destination plate shows the Z-shaped pattern of the interleaved transfer of A1 compounds from the four different 96-well source plates to the 384-well destination plate.
◼️ PROTOCOL 2 | Plate Replication - Testing Assay Conditions
The example reformatting protocol #2 illustrates plate replication to rapidly create identical (daughter) plates from one source (mother) plate for testing different assay conditions. The procedure starts from a 96 deep-well source plate containing bioactive compounds of interest, and the aim is to transfer the same volume of compound solution into three 96-well destination plates containing cell cultures. Each destination culture plate is to be tested in a separate condition.
Figure 3: Andrew+ Domino configuration for the protocol "Plate Replication - Testing Assay Conditions" (left) and experiment setup comparison between Andrew+, Pipette+, and manual pipetting (right). Domino positions on the Andrew+ working deck: [1] Tip Insertion System Domino (10-300 μL tips), [2-4] Microplate Domino, [5] Deepwell Microplate Domino. The time required for Andrew+ to complete the protocol is ~9 min using an 8-channel 300 µL electronic pipette.
Protocols
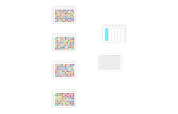
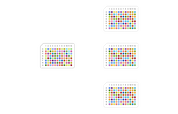
Contact info
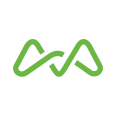
